Research in the EME2Lab sits at the nexus of energy and the environment. We have two overarching goals: (1) identify both short and long-term solutions to the world’s energy needs, and (2) understand and mitigate the impact of energy generation processes on the environment. We have a variety of on-going projects in the lab, incorporating undergraduate, graduate and post-doctoral researchers that look at many facets of energy and its impact on the environment. To implement this vision, our research falls into three main areas:
- Energy’s Environmental Impact: Identifying the environmental impacts and unintended consequences of past and future energy generation;
- Alternative Energy Sources: Investigating economically viable and environmentally sustainable alternative energy sources, for both the short and long-range future;
- Sustainable Materials: Developing materials sourced from renewable precursors and greener processes for pollutant remediation and energy applications.
Manipulating Equilibria to Enhance Biofuels
Tuning Liquid Biofuels from Hydrothermal Liquefaction via Exploiting Fundamental Thermodynamics
Hydrothermal liquefaction (HTL) transforms organic wastes into liquid biofuels by processing the waste in water at high temperature and pressure. One of the advantages of HTL over other biomass conversion techniques is that it directly treats wet wastes without an energy-intensive pre-drying step. To date, the majority of HTL research has explored the impact of process conditions on products generated from a range of biomass feedstocks. However, this research approach cannot overcome the primary challenges to widespread application of HTL for biofuels, namely that (1) we cannot accurately control or predict product distributions, resulting in the need for significant downstream upgrading of the biocrude, and (2) the byproduct process water requires considerable treatment; these two challenges combine to make current large-scale HTL economically infeasible. Our new approach to process design, recently funded by a National Science Foundation CAREER award, could enable widespread implementation of HTL for wet waste to biofuel conversion.
Combustion Behaviors of Hydrochars from Wet Biomass Wastes
Converting Wet Biomass Wastes to Solid Fuels via Hydrothermal Carbonization
Hydrothermal carbonization (HTC) is an economical method to convert wet biomass waste streams, including food, dairy, brewery, sludge, and digester wastes, to hydrochar, a carbon-based solid often called bio-coal for its “coal-like” properties. Despite the growing popularity of HTC as a waste conversion scheme – companies across the world are using HTC reactors to treat wet biomasses– we know little about the fundamental combustion properties of this renewable bio-coal. This limits the potential to use hydrochars for clean energy generation. In a National Science Foundation funded project in collaboration with Prof. Jacqueline O’Connor (Penn State Mechanical Engineering), our research teams will collaborate to uncover (1) how hydrochars form and (2) how hydrochars combust. This knowledge is critical to understanding which waste feedstocks and processing conditions optimize hydrochar fuel properties, and what combustor operating conditions maximize energy recovery. The ability to use renewable solid fuels for energy generation can help mitigate climate change and transform the U.S. into a green energy exporter and job opportunity creator.
Re-engineering the Integrated Biorefinery
Biomass as a Fuel Source and Nanotemplate
SEM: Silver nano-mesh resulting from AgNO3 impregnated shallot peel
This project marries two seemingly disparate fields – production of renewable fuels from biomass and fabrication of novel metal oxide nanoparticles – in a simultaneous process. By impregnating biomass with inorganic compounds, followed by heat treatment, we recover syngas, bio-oil, and bio-templated nanoparticles with unique structures and properties. The inorganic compounds act as a catalyst to improve the stability of the bio-oil. The biomass serves as a removable template for nanoparticle growth, creating structures with higher surface areas and properties mimicking nature. This co-production of energy and materials opens new possibilities in the biorefinery concept, not only to capture energy from renewables such as biomass, but also to produce nanomaterials for a range of applications in a more sustainable way. We are working with Prof. Emily Ryan’s group at Boston University use Materials Informatics to uncover appropriate catalysts, and Prof. Roberto Volpe’s group at Queen Mary University to understand in situ changes to biomass structure. This project is supported by the National Science Foundation under grant No. 1933071. Recent Publications Include articles in Fuel, Journal of Analytical and Applied Pyrolysis and Energy Conversion and Management.
Engineering at the Food-Energy-Water Nexus: in situ Upgraded Biofuels and Water Treatment Materials from the Integrated Biorefinery
The integrated biorefinery could produce sustainable, carbon-neutral energy by converting biomass to renewable fuels. However, a significant barrier to using pyrolysis (heating in the absence of oxygen) as a conversion route is the upgrading required to improve the stability and heating value of the bio-oil. The poor quality and low yield, combined with the cost of such upgrading – especially catalyst degradation and recovery – currently limit the widespread adaptation of these biofuels. In a new approach to the integrated biorefinery we can lower the economic barriers to biofuel production and produce heterogeneous sorbent materials to remediate contaminated water by in situ upgrading biofuels from carbonaceous wastes using inexpensive, abundant clay minerals as solid catalysts. This work is currently supported by a generous seed grant from the Eppley Foundation for Research. Recent Publications Include articles in RSC Advances, Journal of Analytical and Applied Pyrolysis, and Fuel.
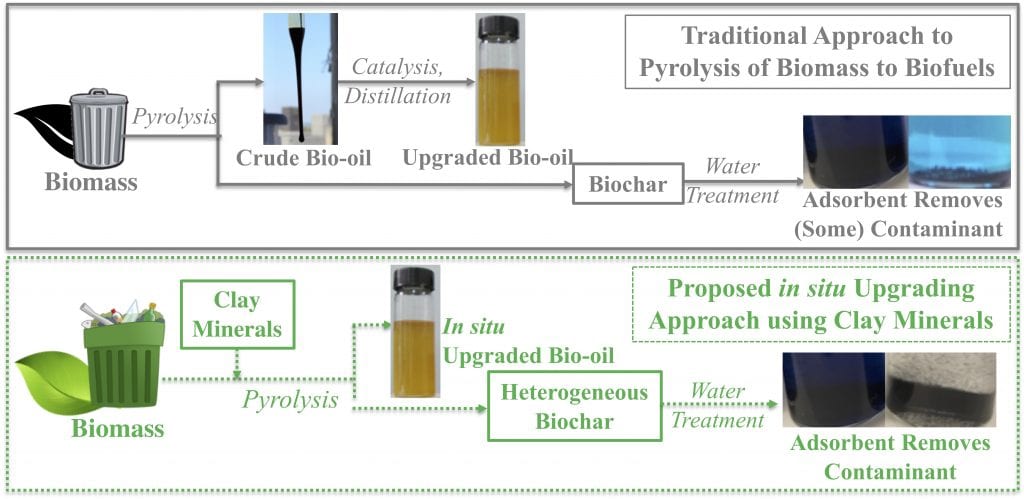
Engineering Hydrochars and Biochars for New York State
Integrated Biorefinery for Conversion of Agriculture & Dairy Waste to Biofuels and Biochars to Protect NYS Water Quality
In Central and Upstate New York, concerns over run-off from dairy and agricultural activities such as grape cultivation, cabbage, corn and hay farming could be assuaged by a new integrated biorefinery. This work, funded by a USDA-NIFA Hatch grant, will design a flexible thermochemical conversion pathway that converts seasonally available biomasses to biofuels and bioproducts. The process design focuses on biomasses specific to NY industries. It will produce soil amendments that act as slow-release fertilizers to mitigate excess nutrient run-off, as well as activated carbon adsorbents to sequester water contaminants, preventing future Hazardous Algal Blooms and protecting drinking water supplies. By converting local farm and food production waste to biofuels, slow release fertilizers and activated carbon adsorbents we can: 1) lower resource consumption; 2) enhance nutrient use efficiency; 3) increase crop yields; 4) improve renewable energy deployment; 5) lessen anthropogenic environmental impacts of industrial agriculture and 6) protect and enhance drinking and recreational water quality across the state. This complements prior work by co-PI Lehmann on pyrolysis of only manure to produce slow-release fertilizers. The proposed work advances the field by looking at impacts of combined feedstocks and processing conditions to produce optimized fuels and materials in an integrated process. Recent publications from this work include an article in Biomass and Bioenergy and Bioresource Technology and ACS Sustainable Chemistry and Engineering.
Efficient Designs for Efficient Materials
Systematic Design of Porous Heterogeneous Hierarchical Materials and Structures to Optimize Reactive Transport Processes
Porous heterogeneous hierarchical engineered materials have a ubiquitous presence across applications as varied as antibacterial agents for biomedical applications, catalysts for fuel upgrading, sorbents for CO2, photocatalysts for H2O treatment, and electrodes for Li-ion batteries. These materials comprise an organic and/or inorganic scaffold designed with multiple levels and distributions of porosities, tortuosities and particle sizes, which are decorated with active sites (often nanoscale inorganic compounds) that can be incorporated during or after “construction” of the scaffold. Despite the myriad of discrete applications, a new integrated computational-experimental approach to the design of these materials that combines materials design with structural design, would force a paradigm shift across these disparate fields. In a new approach to the design of materials, we will work with Prof. Emily Ryan’s group and Prof. Pirooz Vakili’s group to develop a systematic process to design, test, and validate porous heterogeneous hierarchical materials for renewable fuel upgrading.This project is supported by the National Science Foundation.